25/10/2021
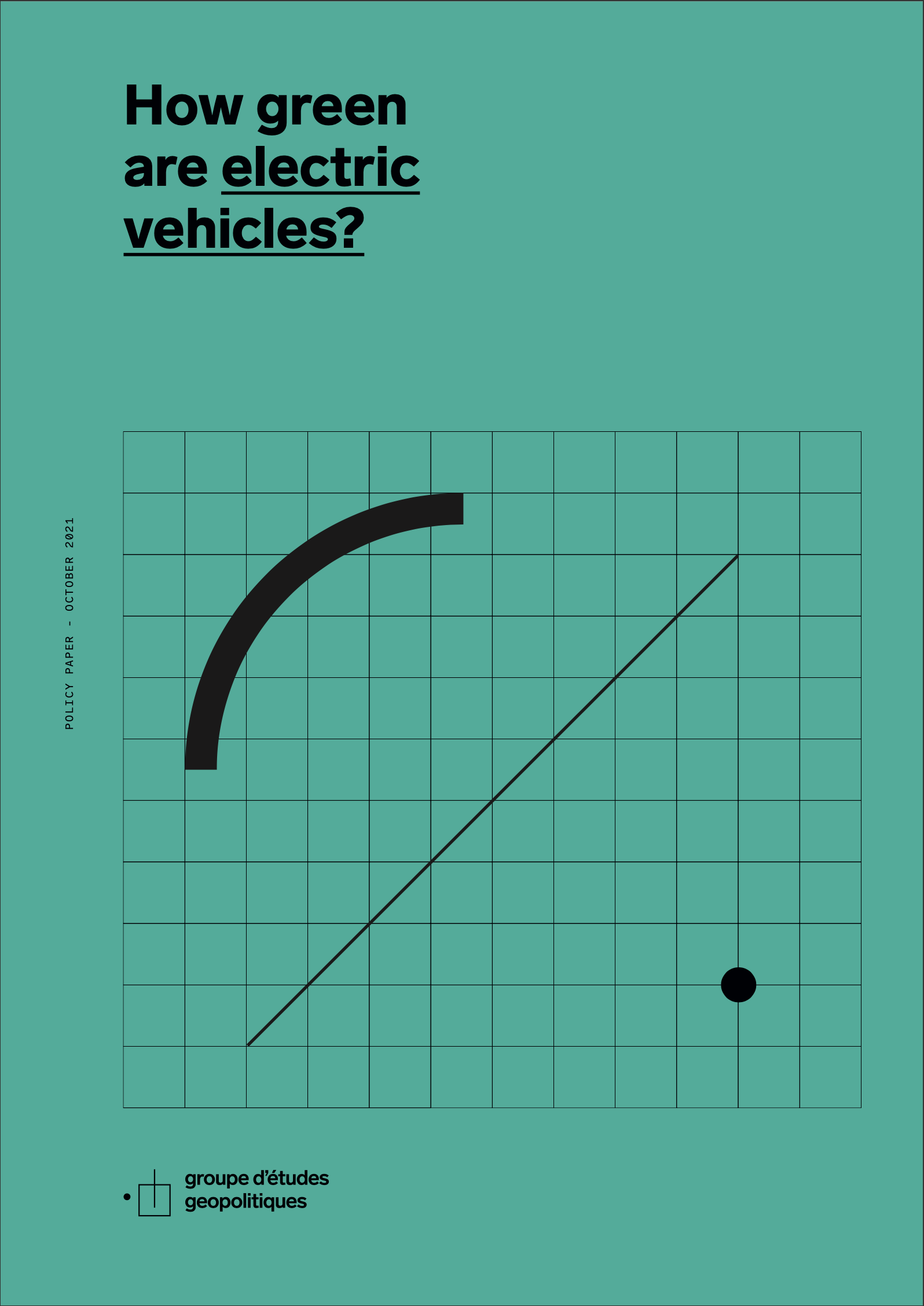
How green are electric vehicles?
Introduction
Globally, transport is responsible for 24 percent of CO2 emissions from fuel combustion 1 . Hence, decarbonisation scenarios (e.g., IEA 2 , IRENA 3 , WEF 4 ) regularly stress the importance of electrifying the transport sector to achieve global climate targets. Although the vast majority of life cycle assessments attribute electric vehicles (EVs) with less life cycle greenhouse gas (GHG) emissions than conventional combustion engine vehicles (see Figure 1), some studies, for example, by the German General Automobile Club 5 or the ifo Institute for Economic Research 6 , disagree. A common second criticism of EVs focuses on the resource-intense and environmentally damaging mineral mining and battery manufacturing processes. To objectively inform policy, it thus appears important to dissect existing life cycle assessments of EVs and identify potential future trends in the different stages of the vehicle life cycle, especially for batteries.
How do electric vehicles compare to conventional ones in terms of life cycle emissions?
The life cycle of an EV consists of two parts: the vehicle cycle and the fuel cycle. The vehicle cycle includes the extraction of materials, production of battery, powertrain and vehicle glider, and maintenance of these parts. Additionally, repurposing or recycling could potentially occur at the end-of-life stage of an EV. The fuel cycle includes the production and consumption of electricity during the use of the EV. In July 2021, the ICCT 7 carried out a life cycle assessment (LCA) comparison of battery electric vehicles (BEVs) and internal combustion engine vehicles (ICEVs), displaying the life cycle GHG emission contributions of the different life cycle steps (Figure 1).
Figure 1: Life cycle GHG emissions of a medium-sized BEV and ICEV registered in 2021 (in g CO2 eq./km)
Source: ICCT, 2021 8 .
As evident, emissions from vehicle manufacture are fairly powertrain-agnostic. The main differences between ICEVs and BEVs emerge at the battery manufacture, which only impacts the life cycle emissions of EVs, and in the fuel cycle. The latter’s GHG emissions are highly dependent on the emission intensity of the fuel and electricity production and consumption.
ICEVs, on the one hand, might reduce their life cycle emissions by changing their fuel composition or achieving better combustion efficiencies. The life cycle emissions of EVs, on the other hand, decrease with lower emission intensities of electricity generation. As ICEVs and gasoline production from fossil fuels are already mature technologies, future potential efficiency improvements are small compared to the potential ones in the fairly nascent EV sector. For example, the impact of future changes in the biofuel blend is only expected to decrease the life cycle emissions of ICEVs by a maximum of 9% 9 for cars registered in 2030.
How do differences in the results of life cycle assessments arise?
The main reason for deviations between different studies lies in the assumptions underpinning LCAs. Different assumptions regarding vehicle lifetimes, technological progress, driving patterns, regional electricity mixes, and procurement locations of vehicle parts contribute to a wide range of LCA results. Figure 2 displays a selection of LCAs dating back to 2015 to showcase the differences resulting from varying assumptions regarding vehicle lifetime and geographical scope.
Figure 2: Life cycle emission reductions of EVs compared to ICEVs (in %)
Source: authors’ elaboration.
Longer vehicle lifetimes clearly increase the emission benefit of EVs compared to ICEVs. Moreover, assessments in different regions or countries show that different regional emission intensities of electricity generation influence the comparison of life cycle emissions. For example, the life cycle emission reductions of an EV in Poland are much smaller than the one achieved in Germany or France, according to an assessment by Transport & Environment in 2020 10 . This difference is mainly due to the GHG emission intensity of electricity generation 11 being 751 g CO2 eq./kWh in Poland, while electricity in Germany and France is generated with a GHG emission intensity of 350 g CO2 eq./kWh and 56 g CO2 eq./kWh, respectively. Finally, a recent study 12 estimates that on a global level an average EV would emit less life cycle emissions than an average ICEV, if the emission intensity of electricity generation is below 1100 g CO2 eq./kWh (estimations are based on a 150.000 km lifetime).
Although the overwhelming majority of recent LCAs attribute EVs with significantly lower lifetime GHG emissions than ICEVs, EVs are not causing zero GHG emissions during their lifetime. While the impact of the electricity mix has already been displayed and governments have implemented policies to achieve the decarbonisation of the mix, the impact of improvements during the life of an EV battery has largely been in the shadow of electricity mix decarbonisation. Moreover, identifying and acting on key levers in the battery life cycle might not only reduce GHG emissions but might also decrease import dependencies, human rights issues, and further environmental hazards.
What is the life cycle of an EV battery?
Currently, lithium-ion batteries are used in EVs, even though other, more efficient, battery technologies might reach commercial and technical viability in the future (e.g., lithium-metal solid-state batteries 13 or lithium-sulphur batteries 14 ). Due to the uncertainty of technological progress, only lithium-ion batteries are considered here.
During the first step of the battery life cycle, the battery is manufactured by combining several hundred individual lithium-ion cells. These individual cells all consist of a cathode, made from a combination of lithium, nickel, manganese, cobalt, and aluminium, and an anode, usually made of graphite. Additionally, a battery also includes a separator and electrolyte. During the manufacture of lithium-ion batteries, GHG emissions mainly emerge from the emission-intensive mining and refining of the cathode minerals. Moreover, the battery manufacture causes significant emissions as the battery assembly must be carried out in non-humid, climate-controlled rooms 15 .
The mining of the cathode minerals is also associated with a wide range of social and environmental issues, for example, highlighted by the IEA 16 . As significant parts of these minerals are mined in Russia or the Democratic Republic of Congo, environmental or human rights oversight is difficult to guarantee. These risks are especially prevalent for EVs, as one vehicle requires over 200 kg of these minerals, more than six times the amount needed for an ICEV 17 .
Furthermore, most of the cathode minerals are currently imported into the EU, posing significant supply risks for lithium and cobalt. Supply issues might only increase in the future, as the IEA forecasts mineral demand to increase 30-fold until 2040 18 in a Sustainable Development Scenario.
After the manufacture and assembly, the EV battery enters the use phase of its life cycle. While past lithium-ion batteries had lifetimes shorter than the ones of passenger cars, new technological developments have extended the lifetime of EV batteries to surpass vehicle lifetimes, as shown by the ICCT 19 and Earthworks 20 .
Once an EV reaches its end-of-life, there are several pathways for the EV battery.
First, lithium-ion batteries might be disposed of. However, once batteries end in landfills, they might contaminate both water and soil 21 . Second, due to the long lifetime of a lithium-ion battery, they maintain around 80% of their total usable capacity 22 . Hence, batteries might be repurposed in residential or even utility-scale energy storage or other vehicles. The repurposing of an EV battery might extend its lifetime by 5-15 years 23 , hence, reducing the lifetime emissions of an EV. The ICCT estimates that battery second life usage could reduce the emissions stemming from battery manufacture by 42% on a kilometre basis over the entire vehicle life cycle 24 .
Furthermore, recycling lithium-ion batteries provides ample potential to reduce the negative environmental and social effects of EV batteries. While different studies foresee varying shares of mineral demand to be covered by recycled minerals from EVs, they all attribute recycling with a key role in increasing the sustainability of EVs. For example, the IEA estimates that the recycling and reuse of EV batteries could reduce the primary supply requirement of lithium and copper by 5%, cobalt by over 12 percent, and nickel by 7.5 percent by 2040 25 . More optimistic scenarios even indicate that 25 percent of the EV industry’s lithium demand and 35 percent of the cobalt demand could be covered by recycling in 2040 26 if all end-of-life EV batteries are recycled. Jeffrey Brian Straubel, former Tesla CTO and the founder of Redwood Materials, one of the most ambitious lithium-ion battery recyclers in the US, even forecasts that life cycle emissions of EVs can be more than halved if batteries are continually recycled 27 .
However, the current share of lithium-ion batteries recycled globally seems to be around only 5 percent, while almost all lead batteries in ICEVs are recycled 28 . Additionally, increased recycling capacities have only managed to keep up with the increase in demand in the past years 29 . Figure 4 displays the current lithium‑ion battery recycling capacities globally, amounting to around 180 kt per year.
Figure 3: Shares in existing and announced global lithium-ion battery recycling to come online by 2021
Source: IEA, 2021 30 .
How to recycle an EV battery?
Currently, three recycling treatments for EV batteries are established, after discharging, stabilizing, and dismantling the batteries in a first step. First, batteries are mechanically treated by shredding and, afterward, filtering out the minerals to be recovered. However, due to the fairly basic process, this treatment procedure often has to be combined with more refined methods 31 . Companies currently pioneering mechanical treatment include AkkuSer from Finland, Retriev Technologies from the US, and Li-Cycle from Canada. By combining mechanical treatment with hydrometallurgical recovery, Li-Cycle claims to be able to recover more than 95 percent of raw materials 32 in lithium-ion batteries, a number far exceeding current average recovery rates.
More refined recycling methods are pyrometallurgical and hydrometallurgical recovery. While the former employs high temperature smelting to reduce battery components to an alloy, the latter uses aqueous solutions to leach minerals from the cathode. Hydrometallurgical recovery seems to be the method utilized the most currently. While the Chinese company Brunp and the French company Valdi have had success combining mechanical treatment with hydrometallurgical recovery, pyrometallurgical recovery is successfully combined with hydrometallurgical one by Umicore (Belgium), JX Nippon (Japan), and Redwood Materials (United States).
Carmakers are beginning to engage in the recycling and repurposing of EV batteries as well. Nissan utilizes old EV batteries in automated guide vehicles in its factories. Volkswagen is doing the same and, additionally, opened its first recycling plant in Germany. French carmaker Renault already recycles all its EV batteries (currently, about a couple of hundred per year 33 ) in a consortium with French waste management company Veolia and Belgian chemical firm Solvay.
Although the mentioned examples highlight the promising outlook for an EV battery circular economy, current recycling capacities will hardly manage to keep up with the increased supply of end-of-life EV batteries once current and future EVs reach the end of their lifetime. While in 2020, spent lithium-ion batteries from EVs amounted to 1.4 GWh, the IEA 34 estimates this amount to reach over 1300 GWh by 2040.
How to further improve the case for EVs in Europe?
In sum, two are the main levers to further strengthen the case for EVs: advancing the decarbonisation of the electricity mix and increasing battery recycling and repurposing. For example, the ICCT 35 estimates that the full recycling of cathode minerals could reduce emissions stemming from the battery manufacture by 50 percent (however, the emission benefits of recycling are not straightforward to determine due to different recycling processes. Other studies conclude a much smaller emission benefit 36 of recycling). While the decarbonisation of electricity generation is already supported by various policies and targets and has successfully been initiated in the past years, policies promoting the recycling and repurposing of EV batteries are still in their infancy – also in the EU.
In 2018, the European Commission, published a “Strategic Action Plan on Batteries”, in which the circular economy of EV batteries plays an integral role. Moreover, one of the most important EU green industrial policy initiatives 37 , the European Battery Alliance, brings together leading researchers and industry participants with the aim of establishing a sustainable battery value chain in Europe. Furthermore, at the end of 2020, the European Commission has also proposed a new regulation concerning batteries 38 , which is expected to enter into force in 2022. Some of the barriers to the effective recycling and repurposing of EV batteries have already been addressed in this proposal, putting the EU at the global forefront in this field 39 . The only larger jurisdiction to have taken significant action is China 40 , which introduced specific guidelines for discharging, disassembling and storing spent batteries as well as extended producer responsibility. In contrast, the US 41 only introduced landfilling bans in a few states so far but has no overarching framework on the national level. Nonetheless, the EU is still faced with other challenges that need to be resolved.
First, EV batteries are difficult to deconstruct. Additionally, current recycling methods are fairly crude, require large amounts of energy, and are related to safety risks for employees 42 . To combat these issues, the EU proposal includes labelling and information obligations, potentially improving the efficiency and safety of the recycling processes. However, these requirements mostly deal with the chemical contents of EV batteries and do not ease the disassembly process. Hence, obligations regarding battery design for assembly and information requirements, detailing how to efficiently disassemble batteries 43 , could provide further efficiency boosts to the recycling process. For example, the UK-based company Aceleron is already showcasing the possibility of such design measures by producing EV batteries with the disassembly process in mind 44 . These efforts are especially important as future developments in the chemical composition of lithium-ion batteries are expected to further increase the energy requirement of recycling 45 . On a similar note, effective repurposing of EV batteries requires information about the storage conditions and remaining capacity of EV batteries 46 , which are already included in the proposed Battery Directive.
Second, EV batteries at their end-of-life need to be available for recycling or repurposing. The EU proposal already includes a landfilling ban and a collection rate target, starting in 2023 and gradually increasing to 70% by 2030. Furthermore, the EU aims to introduce extended producer responsibility for end-of-life batteries. While these measures might lead to increasing collection rates of end-of-life EV batteries, problems might arise when vehicles are exported at the end of their life in the EU or when vehicles remain in consumer ownership at their end-of-life. One solution to these issues might be battery takeback programs linked to financial incentives. Additionally, while the extended producer responsibility includes the transport of waste EV batteries, the logistics of battery transport are currently subject to a host of regulations. Simplifying compliance with these regulations, while still containing environmental and safety hazards, might increase the share of EV batteries brought back.
Finally, the EU proposal sets recycling efficiency targets and mandates for the minimum recycled content in new EV batteries. These targets are also being supported by R&D funding, especially through Horizon 2020 projects that have already received around €500 million 47 . However, if commodity prices for battery minerals are too low, economic incentives to invest in recycling capacities and technology improvements might not emerge in time 48 . Hence, subsidies for battery recycling plants or public investments into waste treatment facilities could support the proposed targets.
Considering the prior analysis, there seems to be a case for the EU to build upon the new battery proposal and further increase its efforts to promote the recycling of EV batteries. Such measures not only reduce the life cycle emissions of EVs but also curb the volatility of supply chains and reduce environmental and social issues during mineral extraction. Moreover, establishing a recycling and repurposing value chain in the EU has the potential to create green jobs and thus contribute to the European Green Deal’s central policy aim of turning the green transition into an industrial opportunity for Europe.
Notes
- IEA Transport Available online: https://www.iea.org/topics/transport (accessed on 1 September 2021).
- IEA Net Zero by 2050 – A Roadmap for the Global Energy Sector; International Energy Agency: Paris, 2021
- IRENA Global Energy Transformation: A Roadmap to 2050; International Renewable Energy Agency: Abu Dhabi, 2019
- Ben Dror, M.; Loane, M.; Lopez, S. Electric Fleets Can Fuel Decarbonisation Efforts. Here’s How; World Economic Forum: Geneva, 2021
- Jungmeier, G.; Canella, L.; Pucker-Singer, J.; Beerman, M. Geschätzte Treibhausgasemissionen Und Primärenergieverbrauch in Der Lebenszyklusanalyse von Pkw-Basierten Verkehrssystemen; Johanneum Research Life: Graz, 2019
- Bieker, G. A Global Comparison of the Life-Cycle Greenhouse Gas Emissions of Combustion Engine and Electric Passenger Cars | International Council on Clean Transportation; ICCT: Berlin, 2021
- Bieker, G. A Global Comparison of the Life-Cycle Greenhouse Gas Emissions of Combustion Engine and Electric Passenger Cars | International Council on Clean Transportation; ICCT: Berlin, 2021
- Bieker, G. A Global Comparison of the Life-Cycle Greenhouse Gas Emissions of Combustion Engine and Electric Passenger Cars | International Council on Clean Transportation; ICCT: Berlin, 2021
- Transport & Environment How Green Are Electric Cars?; Transport & Environment: Brussels, 2020
- European Environment Agency Greenhouse Gas Emission Intensity of Electricity Generation in Europe Available online: https://www.eea.europa.eu/data-and-maps/indicators/overview-of-the-electricity-production-3/assessment-1 (accessed on 1 September 2021).
- Knobloch, F.; Hanssen, S.V.; Lam, A.; Pollitt, H.; Salas, P.; Chewpreecha, U.; Huijbregts, M.A.J.; Mercure, J.-F. Net Emission Reductions from Electric Cars and Heat Pumps in 59 World Regions over Time. Nat Sustain 2020, 3, 437–447, doi:10.1038/s41893-020-0488-7.
- IEA Global EV Outlook 2020; Global EV Outlook; IEA: Paris, 2020.
- Hall, D.; Lutsey, N. Effects of Battery Manufacturing on Electric Vehicle Life-Cycle Greenhouse Gas Emissions | International Council on Clean Transportation; ICCT, Washington.
- IEA Global EV Outlook 2020; Global EV Outlook; IEA: Paris, 2020.
- IEA The Role of Critical Minerals in Clean Energy Transitions; IEA: Paris, 2021
- IEA The Role of Critical Minerals in Clean Energy Transitions; IEA: Paris, 2021
- IEA The Role of Critical Minerals in Clean Energy Transitions; IEA: Paris, 2021
- Bieker, G. A Global Comparison of the Life-Cycle Greenhouse Gas Emissions of Combustion Engine and Electric Passenger Cars | International Council on Clean Transportation; ICCT: Berlin, 2021
- Dominish, E.; Florin, N.; Wakefield-Rann, R. Reducing New Mining for Electric Vehicle Battery Metals: Responsible Sourcing through Demand Reduction Strategies and Recycling; for Earthworks by the Institute for Sustainable Futures: Sydney, 2019
- Marchant, N. 5 Innovators Making the Electric Vehicle Battery More Sustainable Available online: https://www.weforum.org/agenda/2021/05/electric-vehicle-battery-recycling-circular-economy/ (accessed on 1 September 2021).
- IEA The Role of Critical Minerals in Clean Energy Transitions; IEA: Paris, 2021
- IEA Global EV Outlook 2020; Global EV Outlook; IEA: Paris, 2020
- Hall, D.; Lutsey, N. Effects of Battery Manufacturing on Electric Vehicle Life-Cycle Greenhouse Gas Emissions | International Council on Clean Transportation; ICCT, Washington
- IEA The Role of Critical Minerals in Clean Energy Transitions; IEA: Paris, 2021
- Dominish, E.; Florin, N.; Wakefield-Rann, R. Reducing New Mining for Electric Vehicle Battery Metals: Responsible Sourcing through Demand Reduction Strategies and Recycling; for Earthworks by the Institute for Sustainable Futures: Sydney, 2019
- McGee, P.; Sanderson, H. Electric Vehicles: Recycled Batteries and the Search for a Circular Economy. Financial Times 2021.
- Marchant, N. 5 Innovators Making the Electric Vehicle Battery More Sustainable Available online: https://www.weforum.org/agenda/2021/05/electric-vehicle-battery-recycling-circular-economy/ (accessed on 1 September 2021).
- IEA The Role of Critical Minerals in Clean Energy Transitions; IEA: Paris, 2021.
- IEA The Role of Critical Minerals in Clean Energy Transitions; IEA: Paris, 2021
- IEA The Role of Critical Minerals in Clean Energy Transitions; IEA: Paris, 2021
- Marchant, N. 5 Innovators Making the Electric Vehicle Battery More Sustainable Available online: https://www.weforum.org/agenda/2021/05/electric-vehicle-battery-recycling-circular-economy/ (accessed on 1 September 2021).
- Woollacott, E. Electric Cars: What Will Happen to All the Dead Batteries? BBC News 2021.
- IEA The Role of Critical Minerals in Clean Energy Transitions; IEA: Paris, 2021
- Bieker, G. A Global Comparison of the Life-Cycle Greenhouse Gas Emissions of Combustion Engine and Electric Passenger Cars | International Council on Clean Transportation; ICCT: Berlin, 2021
- IEA Global EV Outlook 2020; Global EV Outlook; IEA: Paris, 2020
- Tagliapietra, S.; Veugelers, R. A Green Industrial Policy for Europe | Bruegel 2020.
- European Commission Proposal for a REGULATION OF THE EUROPEAN PARLIAMENT AND OF THE COUNCIL Concerning Batteries and Waste Batteries, Repealing Directive 2006/66/EC and Amending Regulation (EU) No 2019/1020; European Commission: Brussels, 2020
- Dominish, E.; Florin, N.; Wakefield-Rann, R. Reducing New Mining for Electric Vehicle Battery Metals: Responsible Sourcing through Demand Reduction Strategies and Recycling; for Earthworks by the Institute for Sustainable Futures: Sydney, 2019
- IEA The Role of Critical Minerals in Clean Energy Transitions; IEA: Paris, 2021
- IEA Global EV Outlook 2020; Global EV Outlook; IEA: Paris, 2020
- Woollacott, E. Electric Cars: What Will Happen to All the Dead Batteries? BBC News 2021.
- WEF A Vision for a Sustainable Battery Value Chain in 2030; World Economic Forum: Geneva, 2019
- Marchant, N. 5 Innovators Making the Electric Vehicle Battery More Sustainable Available online: https://www.weforum.org/agenda/2021/05/electric-vehicle-battery-recycling-circular-economy/ (accessed on 1 September 2021).
- IEA The Role of Critical Minerals in Clean Energy Transitions; IEA: Paris, 2021
- IEA The Role of Critical Minerals in Clean Energy Transitions; IEA: Paris, 2021
- Dominish, E.; Florin, N.; Wakefield-Rann, R. Reducing New Mining for Electric Vehicle Battery Metals: Responsible Sourcing through Demand Reduction Strategies and Recycling; for Earthworks by the Institute for Sustainable Futures: Sydney, 2019
- IEA Global EV Outlook 2020; Global EV Outlook; IEA: Paris, 2020
citer l'article
Simone Tagliapietra, Victor Vorsatz, How green are electric vehicles?, Oct 2021,